DC sputtering and DC magnetron sputtering are both techniques used for the deposition of thin films.
The primary difference between these two techniques lies in the type of voltage applied to the target material.
4 Key Differences Between DC Sputtering and DC Magnetron Sputtering
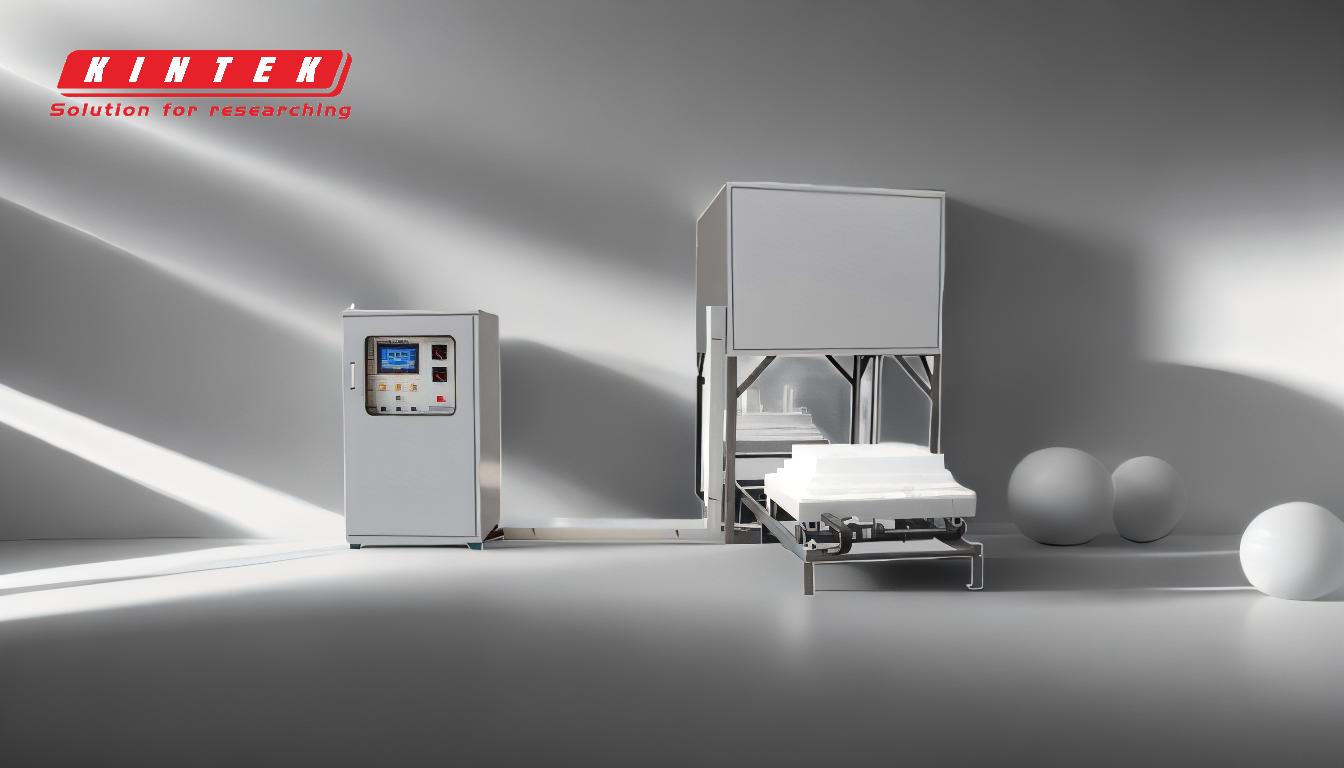
1. Voltage Application
In DC sputtering, a constant voltage is applied to the target material.
This technique is preferred for electrically conductive target materials due to its low cost and high level of control.
DC sputtering involves the use of anodes and cathodes to generate a plasma environment, along with the use of inert gases and optimized sputtering power.
It allows for high deposition rates and precise control over the deposition process.
2. Plasma Efficiency
On the other hand, DC magnetron sputtering involves a vacuum chamber containing the target material parallel to the target substrate.
It is similar to DC sputtering in terms of the constant voltage applied to the target.
However, the use of a magnetron in DC magnetron sputtering allows for a more efficient and concentrated plasma discharge.
This results in higher sputtering rates and improved film quality compared to traditional DC sputtering.
3. Multilayer Deposition
One notable advantage of DC magnetron sputtering is its ability to deposit multilayer structures.
This can be achieved by using multiple targets or rotating the substrate between different targets during the deposition process.
By controlling the deposition parameters and target selection, complex multilayer films with tailored properties can be created for specific applications, such as optical coatings or advanced electronic devices.
4. Application Suitability
Overall, the choice between DC sputtering and DC magnetron sputtering depends on the specific requirements of the thin film deposition process.
DC sputtering is more suitable for electrically conductive target materials, while DC magnetron sputtering offers improved efficiency and the ability to deposit multilayer structures.
Continue exploring, consult our experts
Looking for high-quality thin film deposition techniques? Look no further than KINTEK!
Our laboratory equipment includes state-of-the-art DC magnetron sputtering systems that offer superior film quality and higher deposition rates compared to DC sputtering.
With the added advantage of preventing charge buildup on target surfaces, our equipment is perfect for insulating materials.
Upgrade your thin film deposition process today with KINTEK and experience the difference.
Contact us now!